Anomalous Vascular Dynamics of Nanoworms within Blood Flow
Nanomaterials have been widely used in the design of drug delivery platforms. This work computationally explores the vascular dynamics of nanoworms as drug carriers within blood flow by considering the effects of nanoworm length, stiffness, and local physiological conditions such as hematocrit. We found that nanoworms with length of 8 μm and moderate stiffness are the optimal choice as drug carriers for circulating within normal vascular network due to their lower near wall margination. Compared to those of spherical rigid particles, these nanoworms demonstrate significant demargination behaviors at hematocrit 20%, induced by the local hydrodynamic interactions. Specifically, the interactions between nanoworms and red blood cells create asymmetrical local flow fields, resulting in the demargination of nanoworms. In addition, the flexibility of nanoworms enables them to conform to the deformed shape of red blood cells under shear flow, leading to their high concentration within the core region of vessels. Therefore, the long blood circulation time of nanoworms can be partially attributed to their demargination behaviors and intertwinement with red blood cells. According to these simulation results, tuning the length and stiffness of nanoworms is the key to design drug carries with reduced near wall margination within normal vascular networks and extend their blood circulation time.
https://pubs.acs.org/doi/abs/10.1021/acsbiomaterials.7b00434
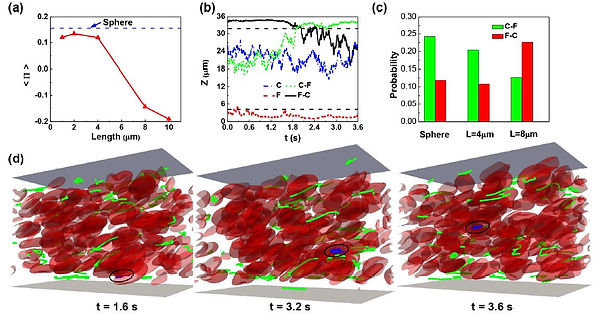
Computational Modeling of Magnetic Particle Margination
within Blood Flow through LAMMPS
We develop a multiscale and multiphysics computational method to investigate the transport of magnetic particles as drug carriers in blood flow under influence of hydrodynamic interaction and external magnetic field. A hybrid coupling method is proposed to handle red blood cell (RBC)-fluid interface (CFI) and magnetic particle-fluid interface (PFI), respectively. Immersed boundary method (IBM)-based velocity coupling is used to account for CFI, which is validated by tank-treading and tumbling behaviors of a single RBC in simple shear flow. While PFI is captured by IBM-based force coupling, which is verified through movement of a single magnetic particle under non-uniform external magnetic field and breakup of a magnetic chain in rotating magnetic field. These two components are seamlessly integrated within the LAMMPS framework, which is a highly parallelized molecular dynamics solver. In addition, we also implement a parallelized Lattice Boltzmann simulator within LAMMPS to handle the fluid flow simulation. Based on the proposed method, we explore the margination behaviors of magnetic particles and magnetic chains within blood flow. We find that the external magnetic field can be used to guide the motion of these magnetic materials and promote their margination to the vascular wall region. Moreover, the scaling performance and speedup test further confirm the high efficiency and robustness of proposed computational method. Therefore, it provides an efficient way to simulate the transport of nanoparticle-based drug carriers within
blood flow in a large scale. The simulation results can be applied in the design of efficient drug delivery vehicles that optimally accumulate within diseased tissue, thus providing better imaging sensitivity, therapeutic efficacy and lower toxicity.


Cell Stiffness Governs its Adhesion Dynamics on
Substrate under Shear Flow
We develop an efficient numerical method to study adhesion dynamics of a single cell on a substrate under shear flow. This method is based on the coupling of Lattice Boltzmann fluid and coarse-grained cell models through immersed boundary method, and a probabilistic model is adopted to capture dynamics of ligand-receptor binding for adhesion. With such a model at hand, a phase diagram of the adhesion dynamics in terms of adhesion strength Ad and stiffness of cell Ca is established. Four types of motion, including free motion, stable rolling, stop-and-go and firm adhesion are found through our numerical simulations, depending on the adhesion strength Ad and stiffness of cell Ca. In addition, demargination behavior of the cell occurs when reducing the adhesion strength Ad in the regime of soft cells (high Ca). Such a demargination behavior is induced by the defomability of cell, resulting in a wall-induced lift force in the shear flow. Lastly, a scaling relation of number of ligand-receptor bonds is obtained under the synergistic effect of Ad and Ca. Present effort provides an robust and efficient way to understand the adhesion dynamics of cells on substrate in shear flow. The obtained results are useful in understanding biological adhesion process and developing adhesion based microfluidic technologies.
Interplay of deformability and adhesion on localization
of elastic micro-particles in blood flow
The margination and adhesion of micro-particles (MPs) have been extensively investigated separately, due to their important applications in biomedical field. However, the cascade process from margination to adhesion should play an important role in the transport of MPs in blood flow. To the best of our knowledge, it has not been explored in the past. Here we numerically study the margination behavior of elastic MPs to blood vessel wall under the interplay of their deformability and adhesion to vessel wall. We use the Lattice Boltzmann method (LBM) and molecular dynamics to solve fluid dynamics and particle (including red blood cells (RBCs) and elastic MPs) dynamics in blood flow, respectively. Additionally, a stochastic ligand-receptor binding model is employed to capture the adhesion behaviors of elastic MPs on the vessel wall. Margination probability is used to quantify the localization of elastic MPs at wall. Two dimensionless numbers are considered to govern the whole process: the capillary number Ca, denoting the ratio of viscous force of fluid flow to elastic interfacial force of MP, and the adhesion number Ad, representing the ratio of adhesion strength to viscous force of fluid flow. We systematically vary them numerically and a margination probability contour is obtained. We find that there exist two optimal regimes favoring high margination probability on the plane $Ca-Ad$. The first regime, namely region I, is that with high adhesion strength and moderate particle stiffness, and the other one, region II, has moderate adhesion strength and large particle stiffness. We conclude that the existence of optimal regimes is governed by the interplay of particle deformability and adhesion strength. The corresponding underlying mechanism is also discussed in detail. There are three major factors to contribute to the localization of MPs: (i) near-wall hydrodynamic collision between RBCs and MPs; (ii) deformation induced migration due to the presence of wall; (iii) adhesive interaction between MPs and the wall. (i) and (iii) promote margination, while (ii) hampers margination. These three factors perform different roles and compete against each other when MPs are located in different regions of the flow channel, i.e. near-wall region. In optimal region I, adhesion outperforms deformation induced migration, and in region II, the deformation induced migration is small compared to the coupling of near-wall hydrodynamic collision and adhesion. The finding of optimal regimes can help understand localization of elastic MPs at wall under the adhesion effect in blood flow. More importantly, our results suggest that softer MP or stronger adhesion is not always the best choice for the localization of MPs.

Shear rate dependent margination of sphere-like, oblate-like and prolate-like micro-particles within blood flow
This study investigates the shear rate dependent margination of micro-particles (MPs) with different shapes in blood flow through numerical simulations. We develop a multiscale computational model to handle the fluid-structure interactions involving in the blood flow simulations. Lattice Boltzmann method (LBM) is used to solve the plasma dynamics and a coarse-grained model is employed to capture the dynamics of red blood cells (RBCs) and MPs. These two solvers are coupled together by immersed boundary method (IBM). The shear rate dependent margination of sphere MP is firstly investigated. We find that margination of sphere MP dramatically increases with the increment of wall shear rate $\dot{\gamma}_\omega$ under 800 $s^{-1}$, induced by the broken of rouleaux in blood flow. However, the margination probability only slowly grows when $\dot{\gamma}_\omega>800\ s^{-1}$. Furthermore, the shape effect of MPs are examined by comparing the margination behaviors of sphere-like, oblate-like and prolate-like MPs under different wall shear rates. We find that the margination of MPs is governed by the interplay of two factors: hydrodynamic collisions with RBCs including collision frequency and collision displacement of MPs, and near wall dynamics. MPs that demonstrate poor performance in one process such as collision frequency may stand out in the other process like near wall dynamics. Specifically, the ellipsoidal MPs (oblate and prolate) with small aspect ratio (AR) outperform those with large AR regardless of the wall shear rate, due to their better performance in both the collision with RBCs and near wall dynamics. Additionally, we find there exists a transition shear rate region $700\ s^{-1}<\dot{\gamma}_\omega<900\ s^{-1}$ for all of these MPs: the margination probability dramatically increases with shear rate below this region and slowly grows above this region, similar to sphere MPs. We further use surface area to volume ratio (SVR) to distinguish different shaped MPs and illustrate their shear rate dependent margination in a contour on shear rate-SVR plane. It is of significance that we can approximately predict the margination of MPs with specific SVR. All these simulation results can be potentially applied to guide the design of micro-drug carriers for biomedical applications.
